A neutrino is the smallest particle in the universe, but what is its exact size? This question has plagued physicists for generations. But, like most problems, the solution can be found by pushing the boundaries.
Physicists from the University of Tsukuba, Kyoto University, and the University of Tokyo in Japan have created a new method for modelling a large chunk of the cosmos to assess the delicate effect of neutrinos on the universe’s evolution.
Since 1930, neutrinos have been a theoretical component of the standard physics model, and since their detection in the mid-1950s, they have also been an experimental cornerstone. These particles should, in theory, have the same mass as photons. However, they not only come in a variety of shapes or ‘flavours,’ but they also oscillate as they move, according to scientists.

As a result, physicists assume that neutrinos must include mass. Furthermore, laboratory investigations have established top parameters for how thick a neutrino may become, putting it at 1/500,000 of the thickness of a single electron. So we have our exact amount somewhere between zero and 1/500,000th of an electron’s mass.
Neutrinos have been a significant part of the Universe since their inception, ejected from the churning vacuum itself during Big Bang. To this day, a neutrally charged background of these neutrino remnants surrounds us, similar to the static hum of residual radiation that we can still detect as a cosmic microwave background. However, there is debate on the fact that massive amounts of relic neutrinos would have impacted the Universe’s nascent structures. It’s difficult to say with certainty what kind of consequence might occur.
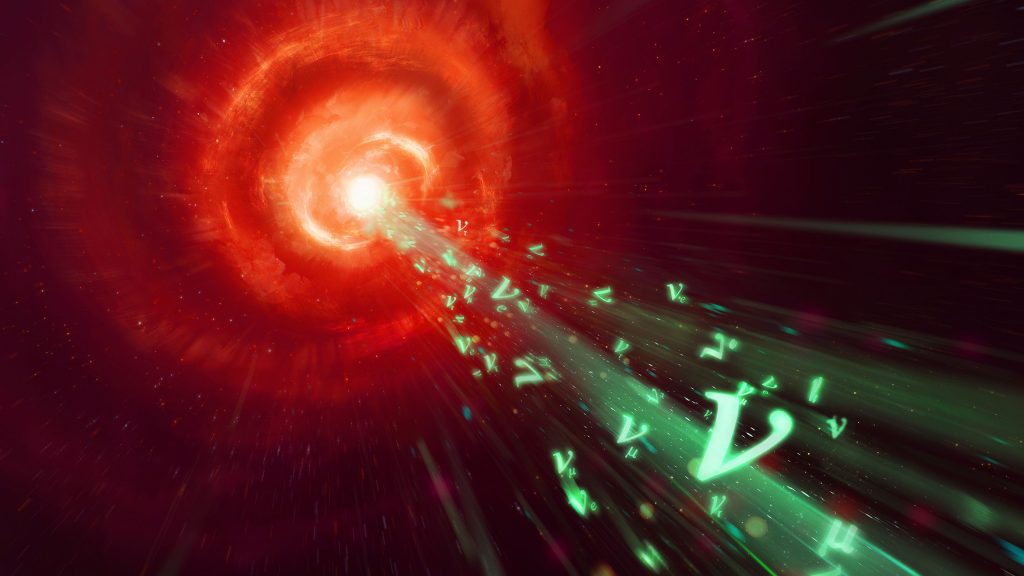
In a typical physics model, for instance, the solar system or a cluster of atoms, you might choose several items, establish their interactions with one another, arrange them in 3D space, and then let a computer analyze what happens over time. ‘N-body’ simulations can help with large-scale simulations. However, they have limits, especially in comparison to quantum physics.
Massive neutrinos, for example, do not act in the same way as classical particles. Since neutrinos only interact with gravity and weak subatomic particles, it’s difficult to say how different forms of neutrinos interacted with the universe’s expansion.
The researchers used a Vlasov simulation equation from plasma physics in this new model during the study. Rather than treating relic neutrinos as discrete objects, the scientists used plasma-based equations to characterize them as a continuous medium.
The experiment was conducted using a supercomputer at Japan’s RIKEN Center for Computational Sciences. It showed how the programme might be used at different scales to provide realistic representations of the structure of much of the observable Universe.
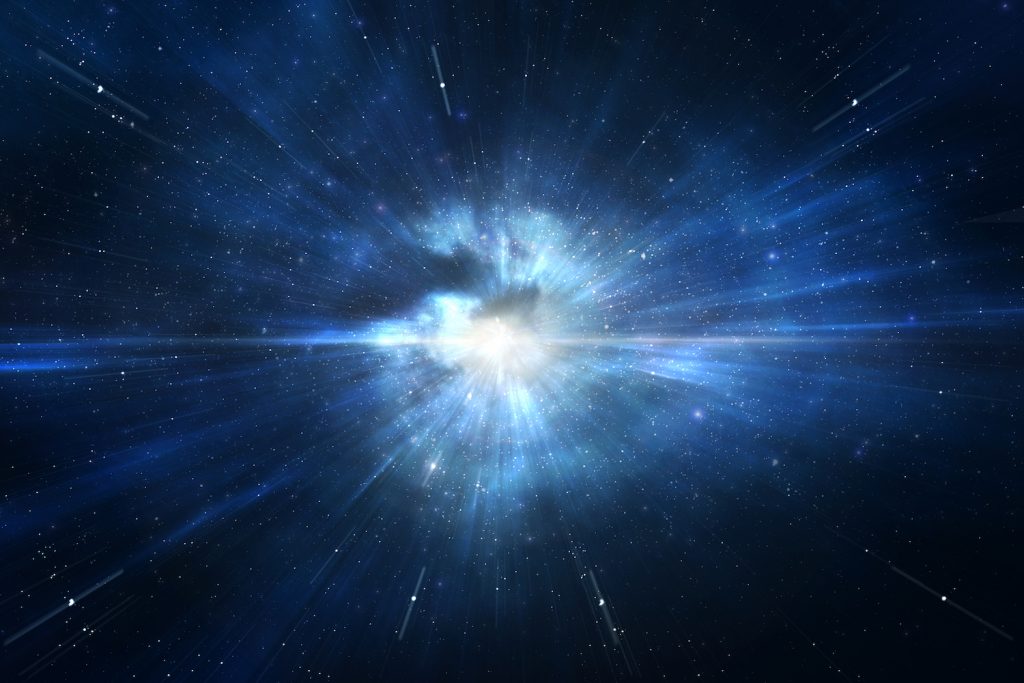
“Our largest simulation self-consistently combines the Vlasov simulation on 400 trillion grids with 330 billion-body calculations, and it accurately reproduces the complex dynamics of cosmic neutrinos,” says the lead author of the study physicist Koji Yoshikawa from the University of Tokyo.
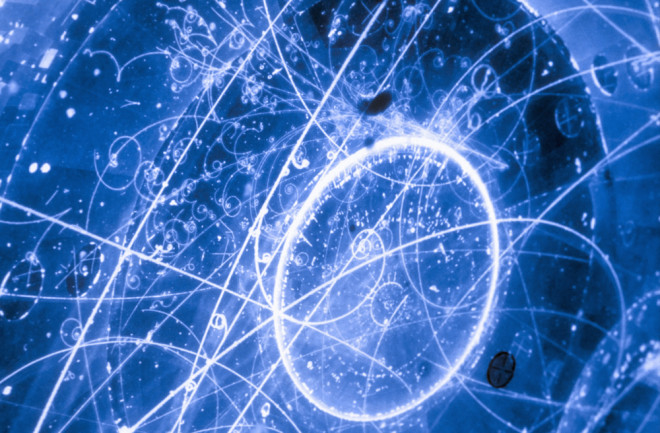
More research will be required to establish a more precise number for the mass of the relic neutrino. Despite this, the team’s achievement has already won them a place as a finalist for the ACM Gordon Bell Prize in 2021. The unique way of large-scale modelling structures could have applications in plasma physics and help physicists discover how much mass a neutrino commands.
This research was published in SC ’21: Proceedings of the International Conference for High-Performance Computing, Networking, Storage and Analysis.