A groundbreaking advancement in diamond synthesis has been achieved by scientists, offering the potential to transform the production of these valuable gemstones. Diamonds naturally originate deep within the Earth’s mantle, subjected to immense pressure and high temperatures.
To replicate these conditions and produce diamonds artificially, methods like high-pressure and high-temperature (HPHT) growth or chemical vapor deposition have been traditionally employed. However, these approaches are hindered by limitations such as the requirement for starter gems and the challenges in sustaining extreme conditions.
A team led by Rodney Ruoff from the Institute for Basic Science in South Korea introduced a novel technique to synthesize diamonds at normal atmospheric pressure, without the need for a starter gem. The method, detailed in a study published in the journal Nature, marks a significant departure from conventional diamond synthesis thinking.
The novel method was a long time in the making. “For over a decade I have been thinking about new ways to grow diamonds, as I thought it might be possible to achieve this in what might be unexpected (per ‘conventional’ thinking) ways,” Ruoff told Live Science by email.
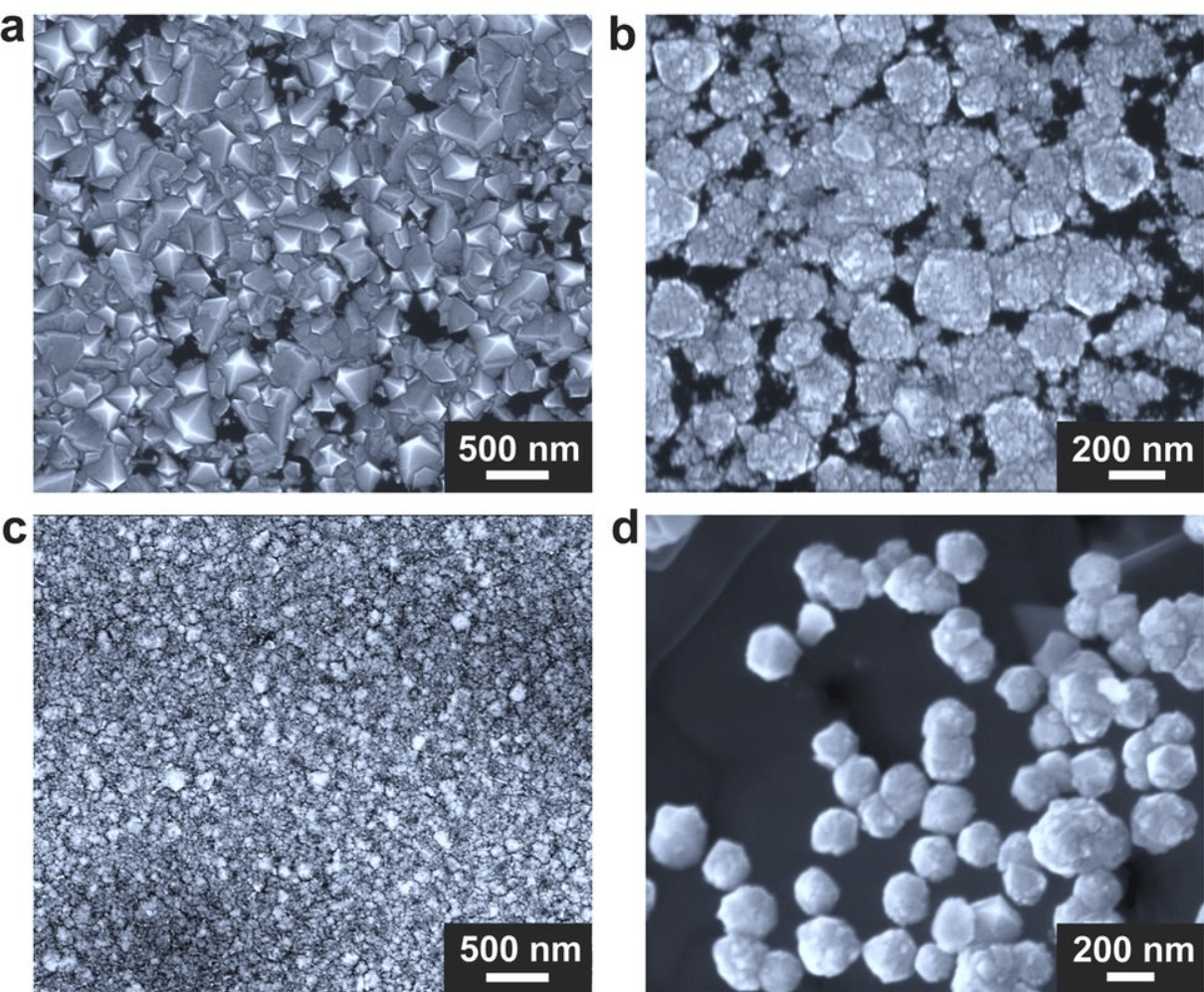
The researchers employed electrically heated gallium with a bit of silicon in a graphite crucible, housed within a chamber maintained at sea-level atmospheric pressure. This setup allowed them to flush superhot, carbon-rich methane gas through the chamber for experimentation.
Through experimentation with different metal and gas concentrations, the team identified a gallium-nickel-iron mixture, along with a pinch of silicon, as optimal for catalyzing diamond growth. Remarkably, diamonds began forming on the crucible’s base within just 15 minutes, with a complete diamond film forming within two and a half hours. Spectroscopic analyses revealed that the resulting diamonds were largely pure, albeit with some silicon impurities.
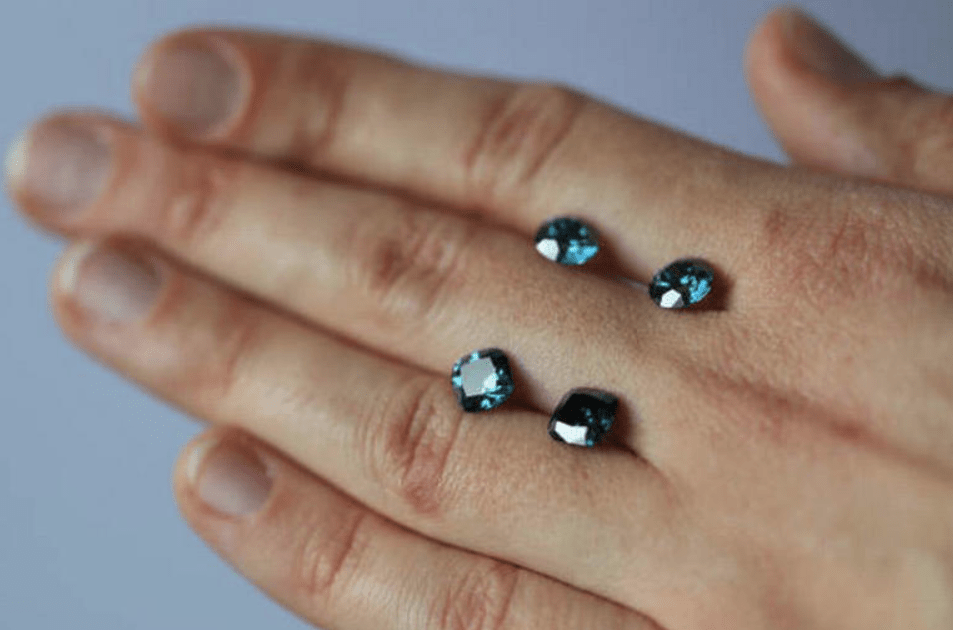
The exact mechanism behind diamond formation in this process remains somewhat unclear, but the researchers suspect that a temperature drop drives carbon from the methane towards the crucible’s center, where it crystallizes into diamond.
Silicon may also play a crucial role as a seed for carbon crystallization. However, one drawback of this new method is the size of the diamonds produced, which are significantly smaller than those grown using HPHT. This limitation currently prevents their use in jewelry.
Nevertheless, there is optimism about the potential applications of diamonds synthesized through this technique, particularly in technological fields such as polishing and drilling. Additionally, the lower pressure requirements of this method could pave the way for scaling up diamond synthesis on a commercial scale. While the full commercial impact remains uncertain, Ruoff suggests that within a year or two, a clearer picture of the potential applications and implications of this breakthrough may emerge.
“In about a year or two, the world might have a clearer picture of things like possible commercial impact,” he added.