Vaccine and drug development, artificial intelligence, transport and logistics, climate science – all these fields will be changed by developing a full-fledged quantum computer. And investment in quantum computers has risen sharply over the past ten years.
Current quantum processors are fairly small, with less than 100 Qubits. This is because bits are the smallest unit of evidence in computing, and qubits are derived from “quantum bits”. Initially, quantum processors were critical in determining the power of quantum computing; the realization of major global applications will likely require processors with more than a million qubits.
However, new research addresses a major issue: How do we go from controlling a few qubits to controlling millions? In research published in Scientific Advances, a new technology is introduced that could provide a possible solution.
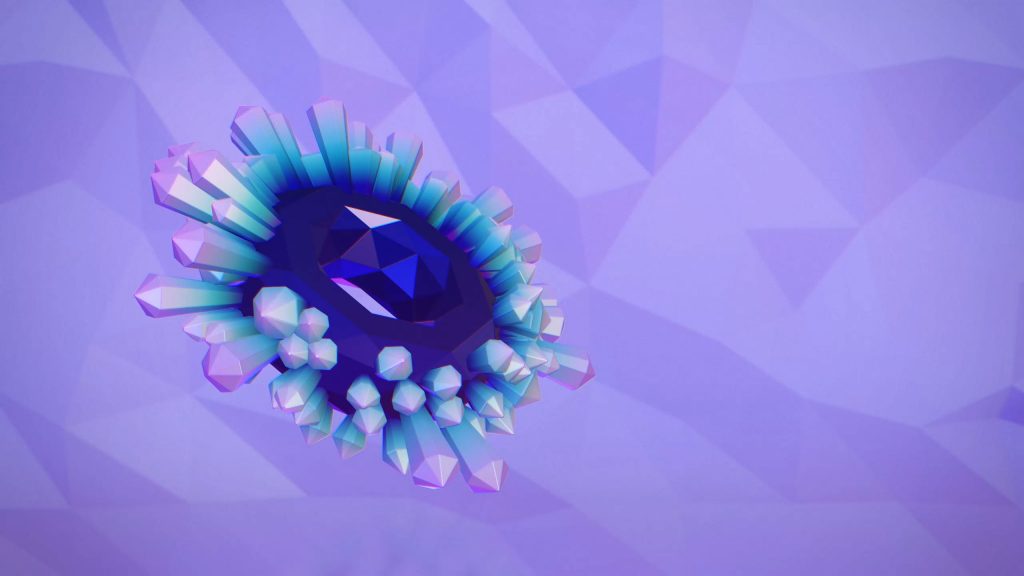
Quantum computers use qubits to store and process quantum information. In contrast to the information bits in classic computers, qubits use nature properties called “superposition” and “entanglement” to do rapid calculations. In addition, unlike a traditional bit, a qubit subsists in two states (that is, 0 and 1) simultaneously. This is formerly known as a superposition state.
The quantum computer from Google had only 53 qubits and was refrigerated to a temperature below -273°C. This extreme temperature melts away the heat, which can lead to errors in the fragile qubits. Though such demonstrations are crucial, the main task is to build quantum processors with additional qubits.
UNSW Sydney is striving to make quantum computers from silicon, the same material used in conventional microchips. A silicon chip contains billions of bits, so the prospect of using this technology to build a quantum computer is somewhat captivating.
In silicon quantum processors, information is stored in individual electrons trapped under small electrodes on the chip surface. Specifically, the qubit is encoded in the electron’s spin. It can be represented as a small compass inside the electron. The compass needle can point north or south, which represents states 0 and 1. To put a qubit in both 0 and 1 states, a control signal must be instructed to the required qubit. In the case of qubits in silicon, this control signal is a microwave field, similar to telephone calls over a 5G network. The microwaves interact with the electron and cause its spin to rotate. Currently, each qubit needs an independent microwave control field. Every cable brings heat that has to be dissipated before it reaches the quantum processor.
With around 50 qubits, this is a bit of an issue; however, manageable. The current refrigerator technology has grown to the cable heat load. However, it is problematic if systems with a million qubits or more are used.
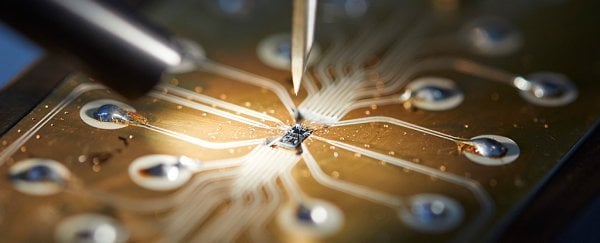
A solution to the challenge of providing millions of spin qubits control signals was proposed in the late 1990s. ??” global control” idea was to send a single microwave control panel over the entire quantum processor.
For more than 20 years, global control in quantum computers existed but like a mere idea. The scientists couldn’t develop a technology integrated into a quantum chip and generate microwave fields with low power.
In this study, it is demonstrated that a so-called dielectric resonator could finally make this possible. The dielectric resonator is a small, transparent crystal that traps microwaves for a short time. Trapping microwaves, also known as resonance, allows them to interact with the spin qubits for longer and reduces the power of the microwaves needed to create the control field.
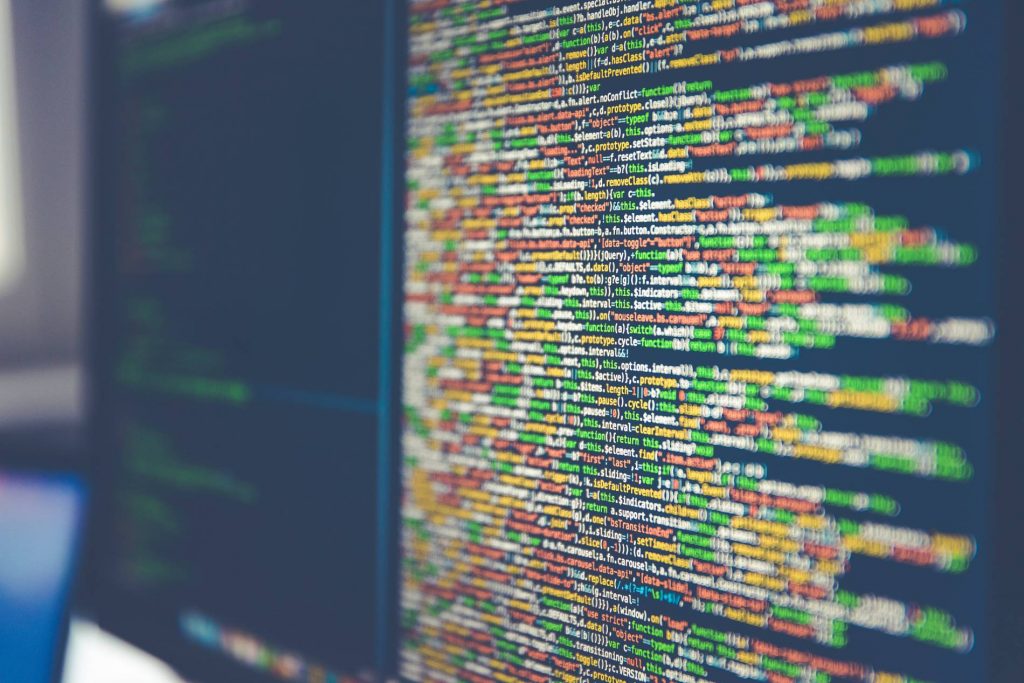
In the recent experiment, the dielectric resonator creates a control field over an area that can contain up to four million qubits. The quantum chip used was a two-qubit device. The research showed that microwaves generated by the crystal could reverse the spin state of each individual.
There is much work to do before this technology is up to the mark. However, this study has successfully succeeded in reversing the state of the qubits, but no arbitrary superimposition states are yet generated.
Experiments are underway to demonstrate this critical ability. In addition, further study on the impact of the dielectric resonator on other aspects of the quantum processor is to be done in the future.